Protein purification using zirconia (ZrO2): Antibodies and His-tagged proteins
We have developed zirconia particles for purifying proteins. These zirconia particles, modified with phosphate groups, exhibit the ability to selectively adsorb antibodies and His-tagged proteins. Due to their cost-effectiveness, these particles can accelerate protein development processes, including the production of medicines and diagnostic pharmaceuticals (Colloid Surf. B 226, 113291 (2023); J. Chromatogr. A 1703, 464112 (2023)).
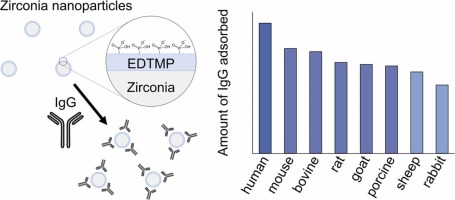
Protein corona (protein-nanoparticle conjugates)
In our research, we aim to understand the properties of protein coronas localized on the surface of nanoparticles. Nanoparticles taken up by living organisms are immediately surface-modified by the physical adsorption of proteins. The protein layer formed on the nanoparticle surface was named "protein corona" by K. A. Dawson in 2007 (Proc. Natl. Acad. Sci. USA 104:2050-2055, 2007), which has been recognized to be associated with the toxicity in recent years. For example, protein coronas inhibit protein-protein interactions, impair the immune system, induce protein fibrillation, and cause structural changes in cell membranes. Because the adsorption of proteins onto nanoparticles, which leads to protein corona formation, is fundamentally in a reversible equilibrium state, the composition of the protein corona dynamically changes over time. The reversibility of adsorption depends on the type of protein, solution environment, and also on the chemical and physical properties of the nanoparticle surface.Experimental studies on the interaction between carbon nanotubes and proteins have been active since 1999, and theoretical studies using molecular dynamics calculations and quantum chemistry calculations have also made progress. We are conducting research on the protein corona of carbon nanotubes and have demonstrated that nanoscale protein aggregates, similar to protein coronas, induce aggregation and disruption of lipid bilayers. This result suggests the unique biophysical properties inherent in protein nanostructures (protein coronas and protein nanoaggregates) (Langmuir 26, 17256-17259, 2010; Langmuir 28, 3887-3895, 2012).
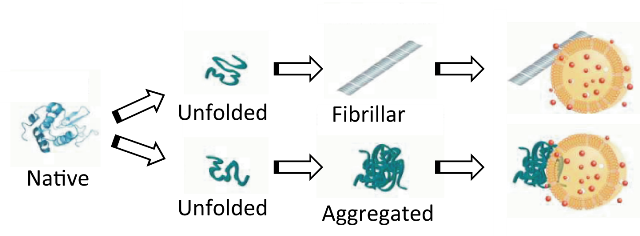
Recently, we demonstrated that nanoscale antibody aggregates increase in size through the adsorption of serum proteins. This dynamic process may be linked to the in vivo behavior of inevitable aggregates of antibody drugs (ACS Appl. Mater. Interfaces 14(29), 32937-32947 (2022)).
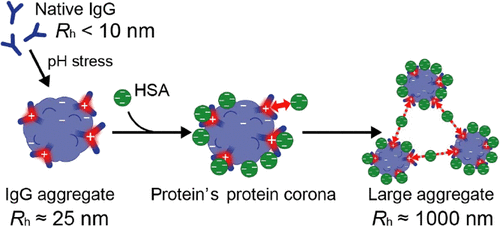
In addition to physical adsorption, oxidative-reductive reactions involving electron transfer are also involved in the adsorption reaction of proteins onto carbon nanotubes. Cysteine, one of the amino acids consisting proteins, reacts with carbon nanotubes to form disulfide bonds (S-S bonds) (Nanoscale 9, 5389-5393 (2017)).
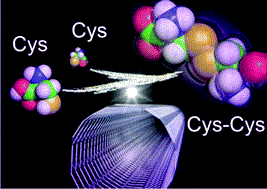
Interestingly, carbon nanotubes appear to promote intramolecular disulfide bonds in proteins (J. Phys. Chem. Lett. 8, 5216-5221 (2017)).
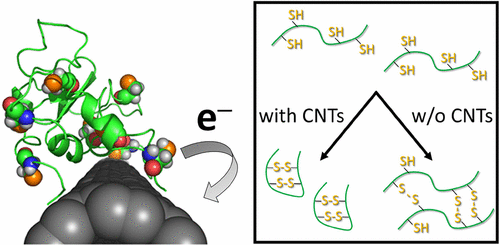
Furthermore, we have also demonstrated that residues of metal catalysts used in the synthesis of carbon nanotubes exacerbate oxidative stress on proteins by promoting oxidative-reductive reactions (ACS Nano 13(2), 1805-1816 (2019)).
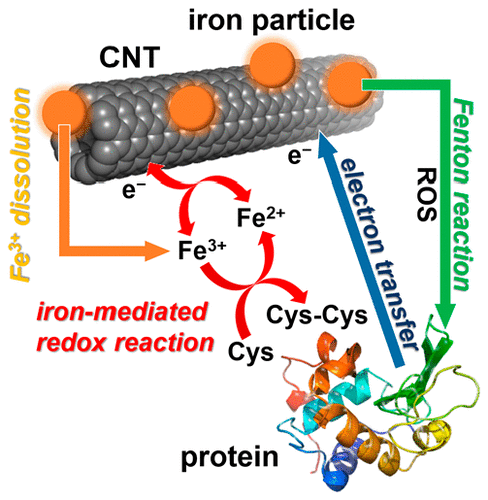
We quantified the binding affinity of proteins to aromatic surfaces such as graphene and carbon nanotubes using molecular dynamics (MD) simulations. The quantified values was named the Aromaphilicity index. The Aromaphilicity index shows a completely different profile from conventional hydrophobicity indices such as Hydropathy. Now, it is possible to predict carbon nanotube binding sites on any protein (ACS Appl. Nano Mater. 2021).
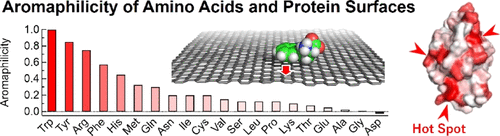
Currently, we are conducting researches on chemical reactions of various biomaterials with carbon nanomaterials (Nanoscale 15(5), 2340-2353 (2023)).
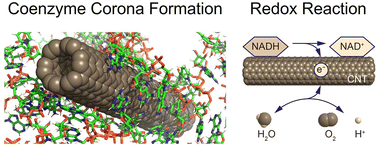
Protein Solution Chemistry: Solubilization, Stabilization & Separation
We aim to understand and control the properties of proteins in solution. We primarily utilize spectroscopic techniques (circular dichroism, infrared spectroscopy, etc.) or thermodynamic methods based on the solubility of amino acids. In addition to solution parameters such as temperature and pH, proteins are influenced by coexisting solutes such as salts and polymers. We aim to optimize these parameters and discover appropriate solution conditions. Furthermore, we also examine solution conditions that enhance protein separation efficiency using columns.
For example, we have demonstrated that the amino acid arginine can suppress protein aggregation and improve the separation efficiency of proteins in multimodal column chromatography. A low molecular weight compound called allantoin has similar functions. Such research in solution chemistry contributes to various fields such as pharmaceuticals and foods (Review: Current Protein and Peptide Science, 20(1), 40-48 (2019)).
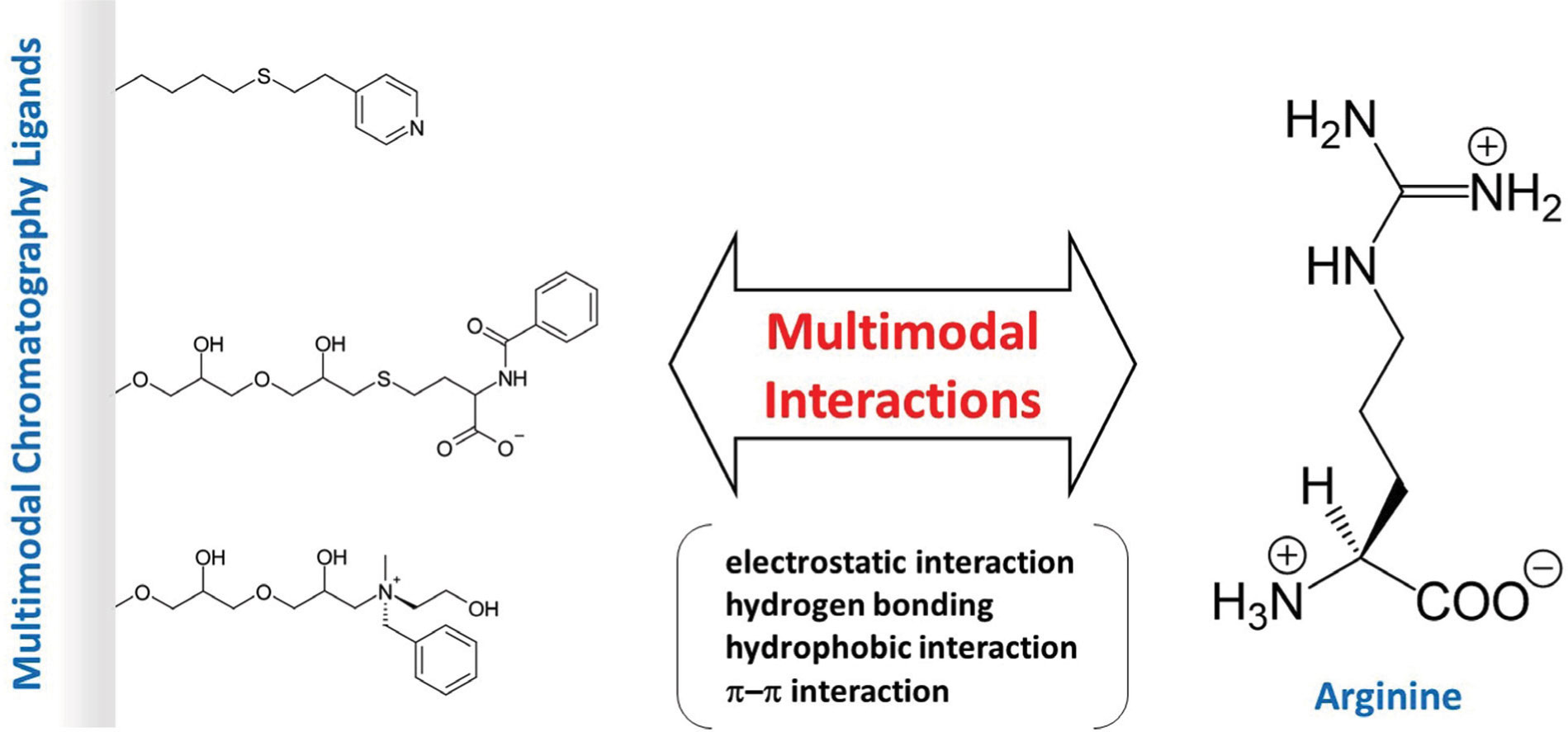
In addition, we are working on techniques to dissolve structural proteins, such as spider silk. Spider silk is renowned as the strongest natural fiber, and utilizing these proteins in composite materials holds promise for sustainable material development. To achieve this, it's crucial to solubilize them for processing. However, they don't readily dissolve in common organic solvents. Therefore, there's a necessity to develop novel solvents and dissolution methods to enhance the solubility of structural proteins. We are studying the solubility of amino acids, the building blocks of proteins, in various organic solvents and developing solvents that are useful for this purpose. (Int. J. Biol. Macromol. 261, 129724 (2024); Int. J. Biol. Macromol. 178, 607-615 (2021)).
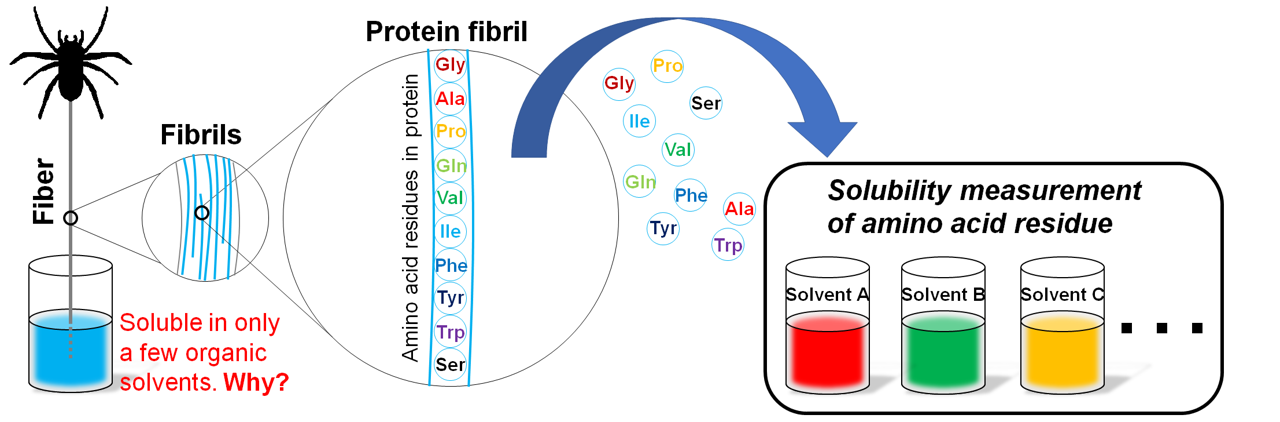
Currently, we are developing silk-carbon nanobute composites using these techniques (JST PRESTO).
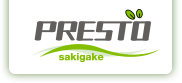
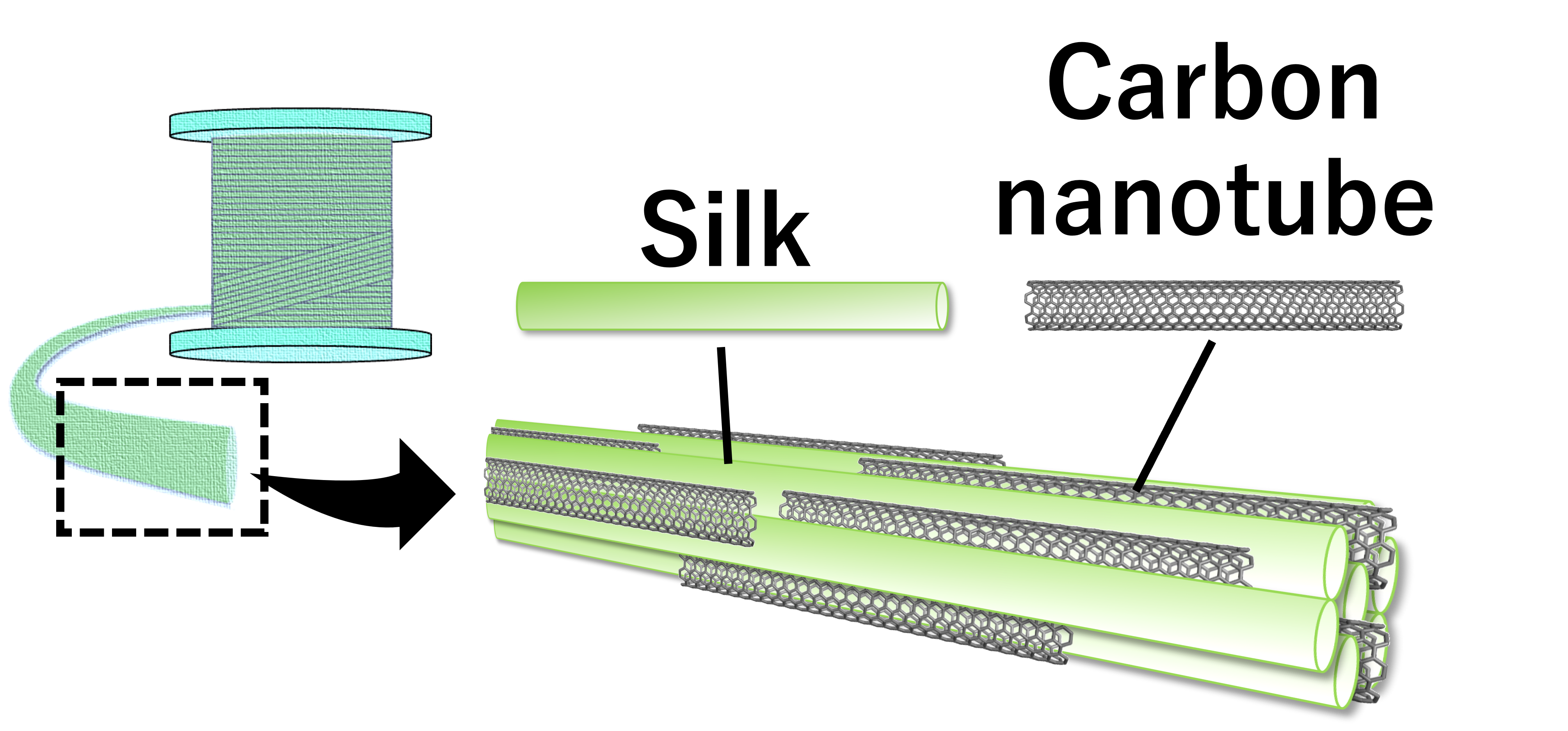
Lecture Notes (Japanese ver.): Protein Dissolution and Aggregation (Free Energy, Aggregation, Denaturation, Crystallization, Additives, Arginine, Hofmeister Series)
Separation of Single-Wall Carbon Nanotubes
Carbon nanotubes (CNTs) possess unique electrical, optical, and mechanical properties, making them promising materials for next-generation nanotechnology. Typically, carbon nanotubes are synthesized as mixtures of different chiralities. However, since CNTs exhibit metallic or semiconductor properties depending on their chirality, it is essential to separate CNTs into metallic and semiconductor types after synthesis for their practical applications. In our group, the metal-semiconductor separation of CNTs was successfully achieved by using gels (Appl. Phys. Express 2, 125002, 2009). The principle behind the metal-semiconductor separation of CNTs is ascribable to thermodynamic and electrochmical reactions (J. Phys. Chem. C, 115, pp 21723-21729, 2011; J. Phys. Chem. C 116, pp 9816-9823, 2012; ACS Nano, 6, pp 10195-10205, 2012; ACS Nano 7, 10285-10295, 2013). Currently, we are developing various materials, devices and composites using these high-quality CNTs.
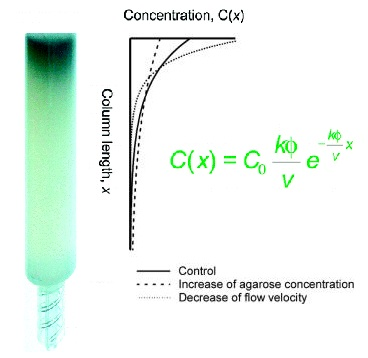
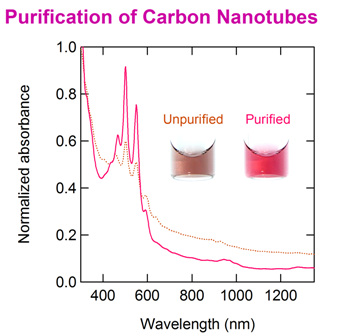
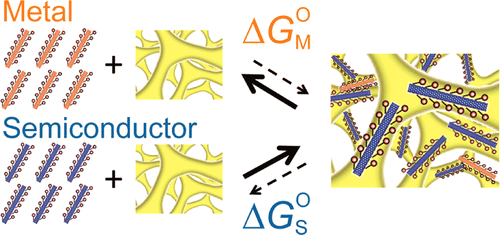
Dispersion of Carbon Nanotubes Using Protein
Carbon nanotubes (CNTs) aggregate in aqueous solution, posing a barrier to their application. Currently, research on dispersing CNTs using biomolecules is actively conducted for applications in the field of biotechnology. Because proteins have lower surfactant properties compared to surfactants, proteins alone cannot efficiently disperse CNTs. We have proposed methods to improve the dispersibility of CNTs in protein solutions using cosolvents and solutes. Typically, alcohols improved the dispersion of CNTs in protein solutions (Chem. Eur. J., 15, 9905-9910, 2009). Urea and guanidine hydrochloride that are known as chaotropic agents were also found to enhance the dispersibility of CNTs (Chem. Eur. J., 16, 12221-12228, 2010). Interestingly, it was observed that the dispersibility of CNTs significantly decreased in high concentration chaotropic solutions. High concentration chaotropes have the property of dissociating CNTs and proteins, leading to the decrease in dispersibility of CNTs. Thus, chaotropic agents can be used to control the dispersibility of CNTs.
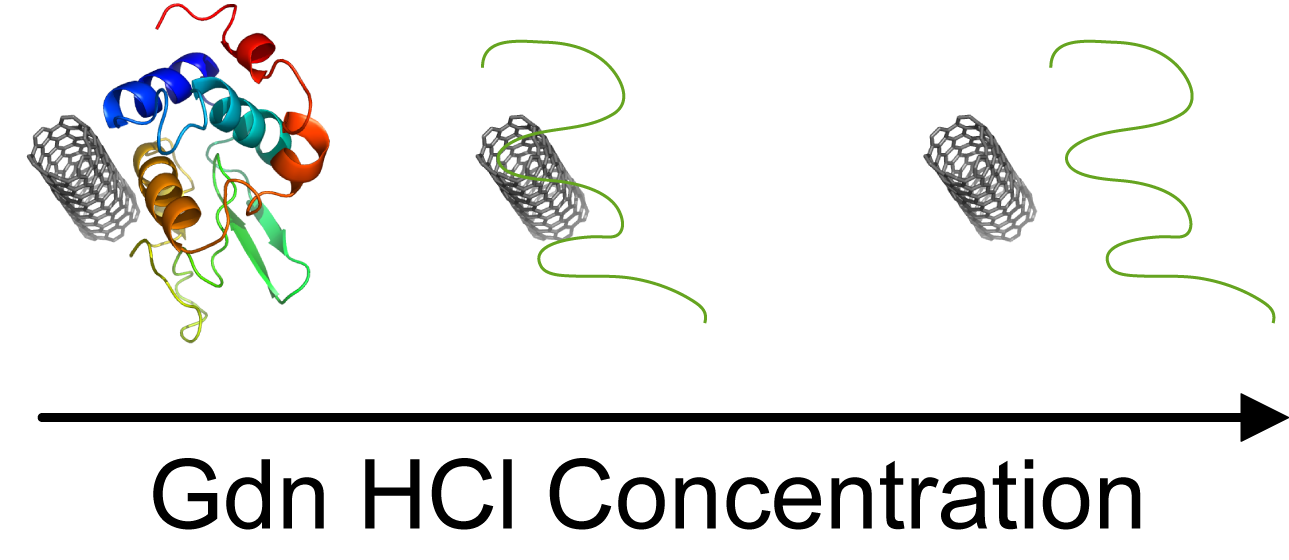
Among the amino acids that consisting proteins, the basic amino acid arginine has been shown to have a high affinity for carbon nanotubes. We have demonstrated that using polyarginine, a homopolypeptide of arginine, efficiently disperses carbon nanotubes (Chem. Eur. J., 20 (17), 4922-4930 (2014)).
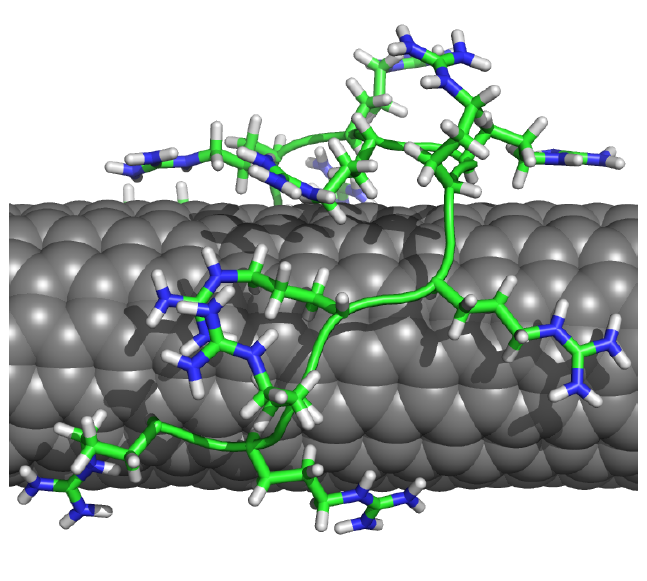
Recently, it has been reported that carbon nanobelts (CNBs) can be chemically synthesized; these materials are the smallest structural units of carbon nanotubes (CNTs). We have found that CNBs can be solubilized using surfactants. While ultrasonication is essential for dispersing carbon nanotubes, it is not necessary for solubilizing CNBs. Solubilized CNBs exhibit zero-Stokes shift fluorescence (Nano Letters 23(23), 11167-11173 (2023)).
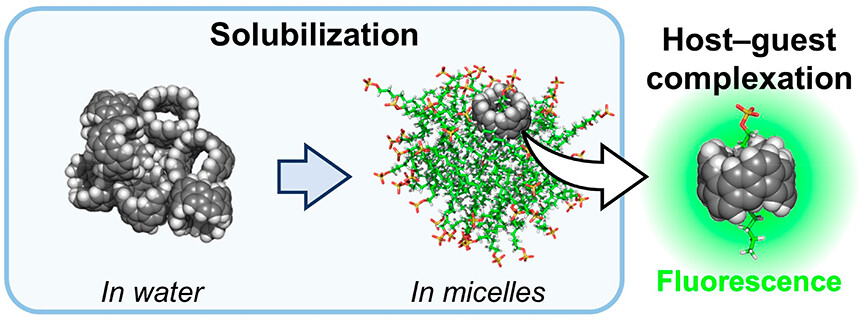