Synthesis and
Thermoelectric
Properties
of Single-Crystal
Cobalt-Based Layerd
Oxides
Introduction
At AIST
Kansai, our group is
active in R&D of
high-performance
thermoelectric
generators (devices for
conversion of heat
energy into electrical
energy), which utilize
oxide materials to
provide stable
functioning for long
periods of time at high
temperatures in air. In
exploring novel
thermoelectric
materials, our focus on
layered materials
composed of alternately
stacked conducting and
insulating layers (Figure
1.) led to a
gfortuitoush success
with the synthesis of a
single-crystal oxide
displaying extremely
high p-type (positive
hole conduction)
thermoelectric
properties, even at high
temperatures in air. The
single crystal
discovered is a layered
oxide with the
composition (Ca2CoO3)xCoO2
(Co-349).
Figure 2 provides a
depiction of the Co-349
crystal structure. The
layered structure
possessed by this oxide
consists of two units
alternately piled one on
top of the other. One of
these units is a CoO2layer
with single Co atoms
surrounded by six O
atoms oriented as
edge-sharing octahedra.
The other is a Ca2CoO3
layer possessing a
rock-salt structure.
What follows is a report
on the method of
synthesis and
thermoelectric
properties of Co-349
single crystals.
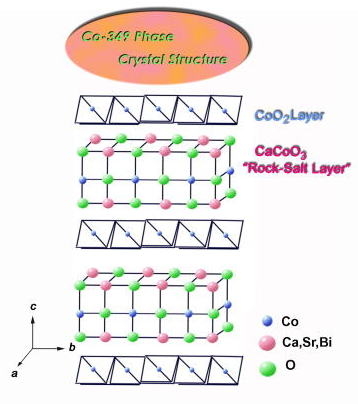 Fig.1 Fig.2
Preparation
Co-349 single
crystals were grown
using a glass annealing
method. (See
Figure 3) Bi2O3,
CaCO3, SrCO3
and Co3O4
powders used as starting
materials were mixed to
achieve an element ratio
of Bi:Ca:Sr:Co =
1:1:1:2, then melted at
1300KC for 30 minutes in
air. The melted raw
materials were poured
and quenched by pressing
between two copper
plates at room
temperature to achieve
glass precursors for
whisker growth.
Annealing of glass
precursors at 920-930KC
for 60-1000 hours under
an oxygen stream yielded
single-crystal
gwiskers.h
Figure 4 presents
photographs of Co-349
whiskers taken using a
scanning electron
microscope (SEM). The
ribbon-like Co-349
whiskers were typically
50-200 m in
width, 1.0-5.0 m
in thickness and 0.1-1.2
mm in length. The broad,
well-grown plane of the
whiskers corresponded to
the ab-plane and
the thickness direction
was parallel to the c-axis.
Typical whisker
composition was Ca1.3Sr0.3Bi0.3Co2Ox
with Sr and Bi
substituting for the Ca
component. The c-axis
length calculated from
x-ray diffraction
patterns was 10.78.
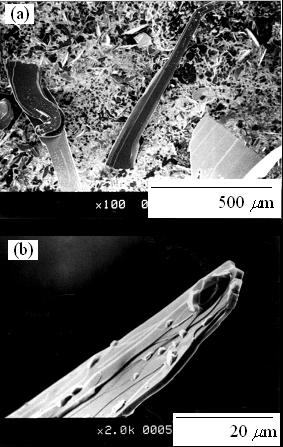
Fig.3
Fig.4
Results
Figure 5 presents a
photograph of a
cross-section of a
Co-349 whisker taken
with a tunneling
electron microscope
(TEM). From this image,
it became clear that the
Co-349 phase possessed a
layered structure
stacked along the c-axis.
Figure 6 shows the
temperature dependency
of the Co-349 whiskerfs
Seebeck coefficient (S)
and electrical
resistivity ().
S is seen to
increase and to
decrease with rising
temperature. At
temperatures of 873 K
and over, S was 200 V/K
or greater. Conversely,
low values of
1.4-1.5 mm were also
recorded.
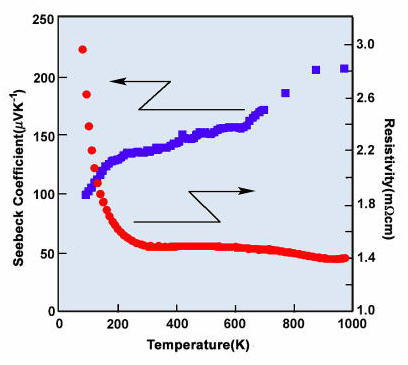
Fig.5
Fig.6
While
the thermal
conductivity()
of the Co-349 whiskers
could not be measured
directly due to the
small size of the
whiskers, of
sintered polycrystalline
material with the same
composition was found to
be at low levels of
around 1.0 Wm-1K-1
at 473-973 K. (See
Figure 7.) This is
thought to stem from
Co-349fs layered
morphology. A misfit, at
the interface between
the CoO2 and
rock-salt layers of the
Co-349 lattice exists,
which causes phonon
scattering thus impeding
lattice thermal
conductivity. The figure
of merit (ZT) of
the Co-349 whisker was
calculated to be 2.7 at
973 K using the
obtained from the
polycrystalline sample.
(See
in
Figure 8.) Generally
speaking, however, the
of a
single-crystal material
should be larger than
that of a sintered
polycrystalline one. At
this juncture, we
derived ZT of
Co-349 whiskers by
calculation of
(= elec+
latt)
using the thermal
conductivity contributed
by the lattice (latt),
which was estimated from
of the
polycrystalline
material, and the
thermal conductivity
contributed by the
charge carriers (elec),
which was calculated
from the
Wiedemann-Franzfs low.
(See
in
Figure 8.) Actual
whisker ZT values
are indicated in the
figure by oblique lines
producing a shaded area
between these two
parameters. As a result,
it became clear that
this oxide possesses
highly outstanding
thermoelectric
properties at high
temperatures in air, as
seen by Co-349 whisker
ZT of 1.2 and over
at temperatures of 873 K
and above.
But
what is it that gives
the Co-349 phase its
exemplary thermoelectric
properties? While the
answer still not
entirely clear,
comparison with Na2CoO4,
which possesses a
structure similar to
Co-349, leads us to
believe that something
in the rock-salt layer
is amplifying the
Seebeck coefficient.
Furthermore, thermal
conductivity is reduced
at layer interfaces. So
what we do know is that
different parts (lattice
and layer interfaces)
within the Co-349
crystal possess
different functions
(reduction of thermal
conductivity and
amplification of Seebeck
coefficient), which
complement each other
superbly, thus realizing
exceptional
thermoelectric
properties. In this
sense, Co-349 is the
very model of AIST
Kansaifs glattice
compositeh approach to
materials design.
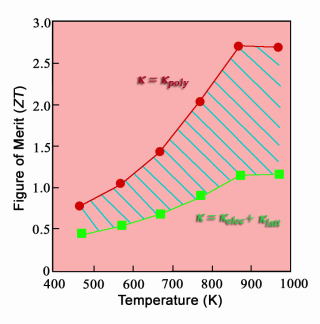 Fig.7
Fig.8
The
Co-349 material
developed thus far
through our research
contains no poisonous or
uncommon elements, and
is chemically stable
even at high
temperatures in air.
This means that it is
highly suited to
thermoelectric power
conversion using
high-temperature waste
heat as an energy
source. In order to
fabricate thermoelectric
generators using Co-349,
from hereon it will be
necessary to develop
large single crystals or
polycrystalline
materials possessing the
same thermoelectric
properties as seen in
the single-crystal
samples. The ZT
of Co-349
polycrystalline samples
prepared by solid-state
sintering was shown to
be one order of
magnitude lower than
that of the whiskers.
The origin of this is
the two-dimensionality
of . (See
Figure 9.) While
along the ab-plane
(ab)
is low, as shown in
Figure 6, along
the c-axis (c)
is 500-1000 times higher
than that of ab.
As a result of this
two-dimensionality, in
polycrystalline samples
is approximately 10
times higher than ab
in the whiskers, and
ZT is thus one order
of magnitude lower. In
order to obtain
polycrystalline
materials with
on the order of that
possessed by the
whiskers, crystal grain
orientation will need to
be aligned along the
ab-plane.
Melt-growth methods or
sintering methods under
uni-axial pressure, such
as hot pressing, are
thought to be an
effective as means of
production for this
purpose.
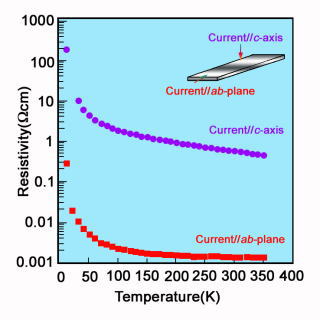
Fig.9
With
the discovery of Co-349,
practical application of
thermoelectric power
conversion utilizing
high-temperature waste
heat, thus far presumed
to be an energy source
confined to the realm of
dreams, has now become a
reality. The objective
from hereon will be to
develop n-type
thermoelectric oxide
materials, and solve
problems related to
technical factors, such
as electrodes, in
fabricating generators
for the purpose of
realizing thermoelectric
power generation from
high-temperature waste
heat.
References
R. Funahashi et al, Jpn.
J. Appl. Phys. 39 (2000)
L1127.
|